In 1934, the oceanographer Alfred C. Redfield took a leap into the unknown. He posited1 that marine nitrogen-fixing organisms — microorganisms that convert nitrogen gas (N2) dissolved in the sea into ‘fixed’ nitrogen compounds that can be used by other organisms to sustain life — might help to explain why the ratio of fixed nitrogen (N) and phosphorus (P) in sea water is similar to their ratio in marine phytoplankton, even though no nitrogen-fixing organisms had been identified at the time. Since then, the search for the regions in which nitrogen fixation occurs, and the role of fixation in controlling the marine nitrogen cycle, have engaged biogeochemists and biologists alike2. But despite much progress, the patterns, rates and limiting factors that control marine nitrogen fixation have remained elusive3.
Read the paper: Convergent estimates of marine nitrogen fixation
In 2007, a study4 that used a geochemical method called the P* approach suggested that a large fraction of marine nitrogen fixation occurs in the eastern tropical Pacific Ocean. This is a region in which depleted oxygen levels in the water column cause fixed nitrogen to be converted back to N2, a process called denitrification. The P* approach analyses the relative abundance of nitrate (the major form of fixed nitrogen) and phosphate in sea water, and estimates the transport and mixing of these nutrients using an ocean-circulation model. In contrast to the results of the P* method, direct measurements of nitrogen-fixation rates suggest that there is a clear spatial separation between nitrogen fixation and denitrification5–7. In a paper in Nature, Wang et al.8 suggest a way of reconciling the results of these two approaches.
The authors pick up many of the threads initially laid out by Redfield and also used in the P* approach. Thus, they use a diagnostic model to work out the sources and sinks of fixed nitrogen and phosphorus implied by the transport and mixing of nitrate and phosphate in sea water, and compare this with the N:P ratio in marine phytoplankton and in the biomass that is exported from the surface to the deep ocean; they assume that any mismatch must be the result of nitrogen fixation or denitrification. But, in contrast to the P* study, Wang and colleagues’ analysis incorporates an observationally constrained model of the ocean’s annual mean circulation9, uses nutrient data from throughout the water column rather than just from the surface, and takes into account the variation of N:P ratios in marine phytoplankton in different regions, rather than assuming a constant ratio. They complement their approach by using a model of ocean biogeochemistry and ecology to investigate the ecological controls on marine nitrogen fixation.
The authors’ diagnostic model infers that most marine nitrogen fixation occurs in the ocean’s subtropical gyres — vast circulation systems that span entire ocean basins at mid-latitudes, and which have low levels of nutrients (Fig. 1). In these regions, nitrogen fixation provides more than 30% of the nitrogen required to fuel the production of biomass and its subsequent export to the deep ocean. The model suggests that, by contrast, very low levels of nitrogen fixation occur in the eastern tropical Pacific and the Arabian Sea, the other tropical regions in which water column denitrification is prevalent.
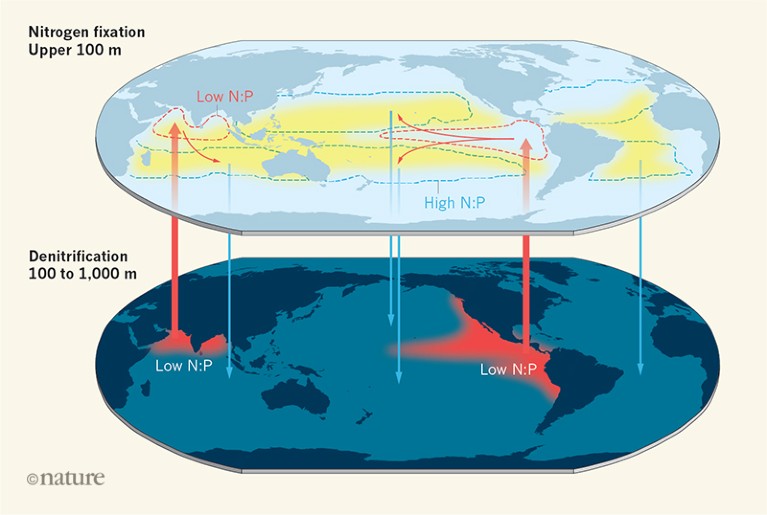
Figure 1 | Links between marine nitrogen fixation and denitrification. Nitrogen gas dissolved in the sea is ‘fixed’ by microorganisms in the upper 100 metres to form nitrogen compounds that are used by other organisms to sustain life. The reverse process — denitrification — occurs at depths of 100–1,000 m in the Indian Ocean and the eastern tropical Pacific Ocean (red areas). Nutrients in waters upwelling from the denitrification zones have very low ratios of nitrogen to phosphorus (N:P). A geochemical method known as the P* approach inferred that most nitrogen fixation occurs close to these denitrification zones4, but Wang and colleagues’ analysis8 now suggests that it occurs mainly in the subtropical gyres (yellow areas) downstream of the tropical upwelling regions. The high levels of nitrogen fixation in the gyres contribute to the formation of biomass that has high N:P ratios, which sinks to the ocean’s interior (blue arrows) and compensates for the loss of nitrogen caused by denitrification.
The shift of nitrogen fixation away from denitrification zones to the subtropical gyres produces a geographical pattern that is much more in line with directly measured fixation rates5 than with the pattern inferred by the P* approach. In particular, the pattern from the diagnostic model is a better match for the low rates measured in the eastern tropical Pacific6 (where the P* approach inferred that fixation rates are highest4), and the high rates observed in the western Pacific7.
The authors argue that the shift is mostly the result of their analysis accounting for the variable N:P ratios in phytoplankton and exported biomass4. Indeed, Wang et al. infer that the N:P ratio of exported biomass varies substantially, from values as high as 21 in the subtropical gyres to as low as 17 in the tropical upwelling regions. Crucially, the authors show that this ratio varies spatially in a similar pattern to that for the ratio of nitrate to phosphate dissolved in sea water. This means that — in contrast to the P* method — their geochemical diagnosis decouples nitrogen fixation from the regions that have low N:P ratios associated with the upwelling of waters that had been subject to denitrification (Fig. 1).
Wang et al. estimate that marine nitrogen fixation adds about 160 (130–220) teragrams of nitrogen per year to the global ocean (1 Tg is 1012 grams). Together with the inputs from rivers and atmospheric sources, about 200 Tg of nitrogen is added to the ocean annually. This is countered by a diagnosed denitrification (a loss) of approximately the same amount. The authors therefore conclude that the global nitrogen budget is almost balanced, and involves input and loss rates that are in line with many previous estimates of the global nitrogen budget10.
The simulations obtained from Wang and co-workers’ biogeochemistry–ecology model suggest that a complex, global mosaic of limitations acts on marine nitrogen fixers. Fixation across most of the Atlantic is limited by the availability of phosphorus, whereas iron is the limiting factor in most of the eastern Pacific. In contrast to the findings of the P* approach4, the effect of the N:P ratio of nutrients seems to be small. Unexpectedly, Wang and co-workers also find that zooplankton strongly affect the abundance and activity of nitrogen fixers: in a simulation in which grazing of nitrogen fixers by zooplankton was strongly curtailed, nitrogen fixation shifted back from the gyres to the eastern tropical Pacific.
The insights gained from the new study are a major step forward in our understanding of the marine nitrogen cycle. In particular, the much larger spatial separation of nitrogen fixation and denitrification compared with the results of the P* approach, and the less-important role of the N:P ratio of nutrients in controlling marine nitrogen fixation, imply that the negative feedback between nitrogen fixation and denitrification is weaker than had been thought. This feedback is key to maintaining the balance between sources and sinks of fixed nitrogen in the ocean10 — a weaker feedback could lead to stronger fluctuations of the fixed-nitrogen inventory in the sea.
Wang and co-workers’ study has some limitations, however. For example, their results could be biased because their models don’t account for seasonal and year-to-year variability — the latter causes large variations in denitrification in the eastern tropical Pacific11. The authors also only partially consider the impact of the substantial perturbation of the marine nitrogen cycle that is associated with increased inputs of fixed nitrogen from the atmosphere and rivers during the Anthropocene (roughly the past two centuries), which has already led to measurable changes in the N:P-nutrient ratios in the ocean12. Modelling studies13 suggest that this perturbation has led to substantial changes in marine nitrogen fixation and denitrification, and to an unbalanced nitrogen budget. Finally, the simulations from the authors’ biogeochemistry–ecology model consider essentially just one type of nitrogen fixer, whereas a diverse range of organisms are capable of nitrogen fixation14. The activity of each group of organisms is probably controlled by different sets of processes.
Nevertheless, Wang et al. have provided strong evidence that nitrogen fixers in the ocean have a well-defined niche in the warm waters of the subtropical gyres — which are generally nutrient-poor, but have enough iron and phosphate to allow nitrogen fixers to compete with other phytoplankton. The new work thus resolves a major discrepancy that has occupied researchers over the past decade3. I am confident, however, that the marine nitrogen cycle has not yet revealed all of its secrets: the areas in which nitrogen fixation occurs might no longer be elusive, but the nitrogen cycle remains a treasure trove for discovery.