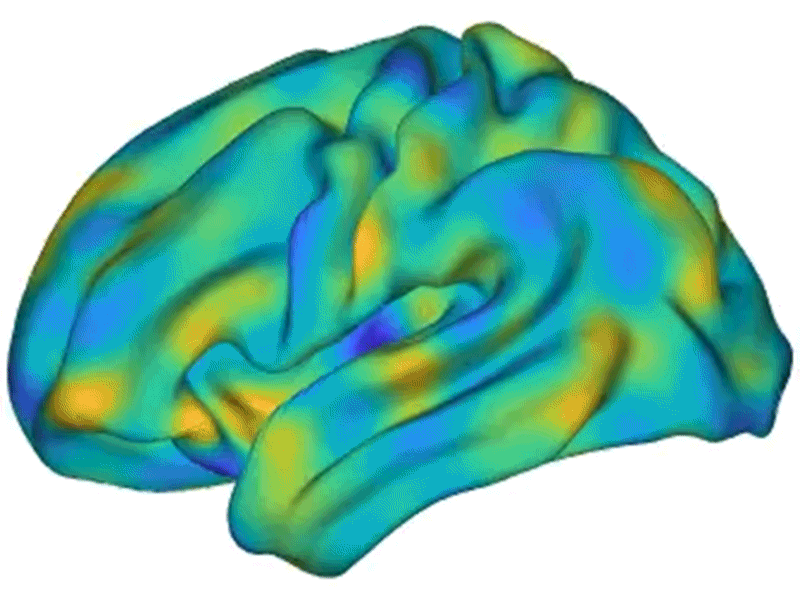
Waves of electrical activity spread across the resting brain in this simulation.J. C. Pang et al./Nature
The wrinkles that give the human brain its familiar walnut-like appearance have a large effect on brain activity, in much the same way that the shape of a bell determines the quality of its sound, a study suggests1. The findings run counter to a commonly held theory about which aspect of brain anatomy drives function.
The study’s authors compared the influence of two components of the brain’s physical structure: the outer folds of the cerebral cortex — the area where most higher-level brain activity occurs — and the connectome, the web of nerves that links distinct regions of the cerebral cortex. The team found that the shape of the outer surface was a better predictor of brainwave data than was the connectome, contrary to the paradigm that the connectome has the dominant role in driving brain activity. “We use concepts from physics and engineering to study how anatomy determines function,” says study co-author James Pang, a physicist at Monash University in Melbourne, Australia. The results were published in Nature on 31 May1.
Brain chatter
‘Exciting’ a neuron makes it fire, which sends a message zipping to other neurons. Excited neurons in the cerebral cortex can communicate their state of excitation to their immediate neighbours on the surface.
But each neuron also has a long filament called an axon that connects it to a faraway region within or beyond the cortex, allowing neurons to send excitatory messages to distant brain cells. In the past two decades, neuroscientists have painstakingly mapped this web of connections — the connectome — in a raft of organisms, including humans.
Famous ‘homunculus’ brain map redrawn to include complex movements
The authors wanted to understand how brain activity is affected by each of the ways in which neuronal excitation can spread: across the brain’s surface or through distant interconnections. To do so, the researchers — who have backgrounds in physics and neuroscience — tapped into the mathematical theory of waves.
Natural phenomena include many types of wave, from the seismic waves that constitute earthquakes to the electromagnetic waves of light. But all of these phenomena are captured by the same simple mathematical equation. This equation enables researchers to calculate the patterns of waves across a surface based exclusively on its geometry. These patterns can be broken down into fundamental components called modes, which are affected by an object’s geometry.
Good vibrations
For waves of vibrations propagating across a sphere, for example, the basic modes include a bulge moving back and forth from the top hemisphere to the bottom one and another travelling from left to right. But a bell lacks a lower hemisphere and therefore has modes different from those of a sphere. An object’s geometry also affects its modes’ characteristic frequencies and relative loudness.
The brain’s neuronal excitation can also come in waves, which can spread across the brain and travel back in periodic oscillations.
The researchers calculated the modes of brainwave propagation for the cortical surface and for the connectome. As a model of the connectome, they used information gathered from diffusion magnetic resonance imaging (MRI), which images brain anatomy. They then looked at data from more than 10,000 records of functional MRI, which images brain activity based on blood flow.
The analysis showed that brainwave modes in the resting brain as well as during a variety of activities — such as during the processing of visual stimuli — were better explained by the surface geometry model than by the connectome one, the researchers found.
David Van Essen, a neuroscientist at Washington University in St. Louis, Missouri, who leads a connectome project, says that the diffusion MRI data the team used has well-documented drawbacks that make the comparison “not fair”. The team should have also looked at brain activity from simple stimuli that activate only local regions of the cortex, according to Van Essen. “It is extremely unlikely that the travelling wave model favoured by the authors could replicate such patterns,” he says.
Pang says that it would be interesting to test their models with such stimuli, and that the analyses he and his co-authors have done so far were a “proof of principle”.
For their study, the authors modelled an idealized brain structure, but the walnut-like convolutions of the cortex are known to vary in shape from one person to the next. The authors’ techniques could help to explore how such variations affect the corresponding modes.