Abstract
Northern peatlands store ~30% of the world’s soil carbon. This carbon sequestration is due to slow decomposition, as illustrated by ancient wooden artefacts and ‘bog bodies’ preserved over millennia. Such artefacts suggest that carbon could be captured externally and stored long term in peat. However, whether such carbon would remain stable following lowered water tables is not known. Here, we show that adding woody litter results in preservation of the exogenous carbon, as well as protection of soil carbon within the host ecosystem from severe drought, as a result of leached polyphenolics. These compounds not only inhibit microbial extracellular and intracellular metabolism but also deprive microbial growth of iron and substrates such as carbon and nitrogen. Our results suggest that this technique harnesses natural ecosystem resilience mechanisms and may have implications for new carbon-farming approaches.
This is a preview of subscription content, access via your institution
Access options
Access Nature and 54 other Nature Portfolio journals
Get Nature+, our best-value online-access subscription
$29.99 / 30 days
cancel any time
Subscribe to this journal
Receive 12 print issues and online access
$209.00 per year
only $17.42 per issue
Buy this article
- Purchase on Springer Link
- Instant access to full article PDF
Prices may be subject to local taxes which are calculated during checkout





Similar content being viewed by others
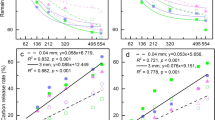
Data availability
The data that support the findings of this study are available from the corresponding author on request. Source data for Figs. 1–5 are included with the paper.
Code availability
The code used to generate Figs. 1–5 can be accessed at https://figshare.com/.
References
Gorham, E. Northern peatlands: role in the carbon cycle and probable responses to climatic warming. Ecol. Appl. 1, 182–195 (1991).
Limpens, J. et al. Peatlands and the carbon cycle: from local processes to global implications—a synthesis. Biogeosciences 5, 1475–1491 (2008).
Holden, J. Peatland hydrology and carbon release: why small-scale process matters. Phil. Trans. R. Soc. Lond. A 363, 2891–2913 (2005).
Bonan, G. B. Forests and climate change: forcings, feedbacks, and the climate benefits of forests. Science 320, 1444–1449 (2008).
Depro, B. M., Murray, B. C., Alig, R. J. & Shanks, A. Public land, timber harvests, and climate mitigation: quantifying carbon sequestration potential on US public timberlands. Ecol. Manag. 255, 1122–1134 (2008).
Pan, Y. et al. A large and persistent carbon sink in the world’s forests. Science 333, 988–993 (2011).
IPCC Climate Change 2014: Impacts, Adaptation, and Vulnerability (eds Field, C. B. et al.) (Cambridge Univ. Press, 2014).
Korner, C. Slow in, rapid out—carbon flux studies and Kyoto targets. Science 300, 1242–1243 (2003).
Freeman, C., Ostle, N. & Kang, H. An enzymic latch on a global carbon store. Nature 409, 149 (2001).
Laiho, R. Decomposition in peatlands: reconciling seemingly contrasting results on the impacts of lowered water levels. Soil Biol. Biochem. 38, 2011–2024 (2006).
Fenner, N. & Freeman, C. Drought-induced carbon loss in peatlands. Nat. Geosci. 4, 895–900 (2011).
Freeman, C., Fenner, N. & Shirsat, A. H. Peatland geoengineering: an alternative approach to terrestrial carbon sequestration. Phil. Trans. R. Soc. Lond. A 370, 4404–4421 (2012).
Fenner, N., Freeman, C. & Reynolds, B. Hydrological effects on the diversity of phenolic degrading bacteria in a peatland: implications for carbon cycling. Soil Biol. Biochem. 37, 1277–1287 (2005).
Wang, H., Richardson, C. J. & Ho, M. Dual controls on carbon loss during drought in peatlands. Nat. Clim. Change 5, 584–588 (2015).
Aloui, F., Ayadi, N., Charrier, F. & Charrier, B. Durability of European oak (Quercus petraea and Quercus robur) against white rot fungi (Coriolus versicolor): relations with phenol extractives. Holz Roh Werkst. 62, 286–290 (2004).
Bragazza, L. et al. Atmospheric nitrogen deposition promotes carbon loss from peat bogs. Proc. Natl Acad. Sci. USA 103, 119386–119389 (2006).
Rasmussen, S., Wolff, C. & Rudolph, H. Compartmentalization of phenolic constituents in Sphagnum. Phytochemistry 38, 35–39 (1995).
Painter, T. J. Lindow Man, Tollund Man and other peat-bog bodies: the preservative and antimicrobial action of sphagnan, a reactive glycuronoglycan with tanning and sequestering properties. Carbohydr. Polym. 15, 123–142 (1991).
Guyette, R. P., Dey, D. C. & Stambaugh, M. C. The temporal distribution and carbon storage of large oak wood in streams and floodplain deposits. Ecosystems 11, 643–653 (2008).
Leifeld, J. & Menichetti, L. The underappreciated potential of peatlands in global climate change mitigation strategies. Nat. Commun. 9, 1071 (2018).
Tian et al. The terrestrial biosphere as a net source of greenhouse gases to the atmosphere. Nature 531, 225–228 (2016).
Freeman, C. et al. Export of dissolved organic carbon from peatlands under elevated carbon dioxide levels. Nature 430, 195–198 (2004).
Goslan, E. H. et al. A comparison of disinfection by-products found in chlorinated and chloraminated drinking waters in Scotland. Water Res. 43, 4698–4706 (2009).
Freeman, C., Lock, M. A., Marxsen, J. & Jones, S. E. Inhibitory effects of high molecular weight dissolved organic matter upon metabolic processes of biofilms from contrasting rivers and streams. Freshw. Biol. 24, 159–166 (1990).
Bonnett, S. A. F., Maltby, E. & Freeman, C. Hydrological legacy determines the type of enzyme inhibition in a peatlands chronosequence. Sci. Rep. 7, 9948 (2017).
Bragazza, L., Parisod, J., Buttler, A. & Bardgett, R. D. Biogeochemical plant–soil microbe feedback in response to climate warming in peatlands. Nat. Clim. Change 3, 273–277 (2012).
Dise, N. Environmental science. Peatland response to global change. Science 326, 810–811 (2009).
Laiho et al. Scots pine litter decomposition along drainage succession and soil nutrient gradients in peatland forests, and the effects of inter-annual weather variation. Soil Biol. Biochem. 36, 1095–1109 (2004).
Williams, C. J., Shingara, E. A. & Yavitt, J. B. Phenol oxidase activity in peatlands in New York State: response to summer drought. Wetlands 20, 416–421 (2000).
Barthelmes, A., Couwenberg, J. & Joosten, H. Slight drainage may enhance peat carbon sequestration in alder carrs. In Proc. International Conference on Carbon in Peatlands (2010).
Dai, J. & Mumper, R. J. Plant phenolics: extraction, analysis and their antioxidant and anticancer properties. Molecules 15, 7313–7352 (2010).
Scalbert, A. Antimicrobial properties of tannins. Phytochemistry 30, 3875–3883 (1991).
Wetzel, R. G. Gradient-dominated ecosystems—sources and regulatory functions of dissolved organic matter in freshwater ecosystems. Hydrobiologia 229, 181–198 (1992).
Dunn, C. & Freeman, C. The role of molecular weight in the enzyme-inhibiting effect of phenolics: the significance in peatland carbon sequestration. Ecol. Eng. 114, 162–166 (2018).
Jones, D. L. & Kielland, K. Soil amino acid turnover dominates the nitrogen flux in permafrost-dominated taiga forest soils. Soil Biol. Biochem. 34, 209–219 (2002).
Van Bodegom, P. M., Broekman, R., Van Dijk, J., Bakker, C. & Aerts, R. Ferrous iron stimulates phenol oxidase activity and organic matter decomposition in waterlogged wetlands. Biogeochem 76, 69–83 (2005).
Wang, Y., Wang, H., He, J.-S. & Feng, X. Iron-mediated soil carbon response to water-table decline in an alpine wetland. Nat. Commun. 8, 1–9 (2017).
Charman, D. Peatlands and Environmental Change (Wiley, 2002).
Wichtmann, W. & Joosten, H. Paludiculture: peat formation and renewable resources from rewetted peatlands. IMCG Newsl. 2007-3, 24–28 (2007).
Worrall, F., et al. A Review of Current Evidence on Carbon Fluxes and Greenhouse Gas Emissions from UK Peatland Report No. 442 (JNCC, 2011).
Wichtmann, W. & Schäfer, A. in Wetlands: Monitoring, Modelling and Management (eds Okruszko, T. et al.) 223–279 (Taylor & Francis, 2007).
Wigley, T. M. L. A. Combined mitigation/geoengineering approach to climate stabilization. Science 314, 452–454 (2006).
Schröder, C., Dahms, T., Paulitz, J., Wichtmann, W. & Wichmann, S. Towards large-scale paludiculture: addressing the challenges of biomass harvesting in wet and rewetted peatlands. Mires Peat 16, 13 (2015).
Prager, A., Barthelmes, A. & Joosten, H. A touch of tropics in temperate mires: on Alder carrs and carbon cycles. Peatl. Int. 2006/2, 26–31 (2006).
Yang, J. S., Nib, J. R., Yuana, H. L. & Wang, E. Biodegradation of three different wood chips by Pseudomonas sp. PKE117. Int. Biodeterior. Biodegrad. 60, 90–95 (2007).
Box, J. D. Investigation of the Folin Ciocalteu reagent for determination of polyphenolic substances in natural waters. Water Res. 17, 511–525 (1983).
Freeman, C., Lock, M. A. & Reynolds, B. Fluxes of carbon dioxide, methane and nitrous oxide from a Welsh peatland following simulation of water table draw-down: potential feed-back to climatic change. Biogeochemistry 19, 51–60 (1993).
IPCC 2013 Supplement to the 2006 IPCC Guidelines for National Greenhouse Gas Inventories: Wetlands (eds Hiraishi, T. et al.) (IPCC, 2014).
Chen, Y. P., Lopez-de-Victoria, G. & Lovell, C. R. Utilization of aromatic compounds as carbon and energy sources during growth and N2-fixation by free-living nitrogen fixing bacteria. Arch. Microbiol. 159, 207–212 (1993).
Freeman, C., Ostle, N. J., Fenner, N. & Kang, H. A regulatory role for phenol oxidase during decomposition in peatlands. Soil Biol. Biochem. 36, 1663–1667 (2004).
Ranneklev, S. B. & Bååth, E. Temperature-driven adaptation of the bacterial community in peat measured by using thymidine and leucine incorporation. Appl. Environ. Microbiol. 67, 1116–1122 (2001).
Claessens, H., Oosterbaan, A., Savill, P. & Rondeux, J. A review of the characteristics of black alder (Alnus glutinosa (L.) Gaertn.) and their implications for silvicultural practices. Forestry 83, 163–175 (2010).
Fenner, N. et al. Elevated CO2 effects on peatland plant community carbon dynamics and DOC production. Ecosystems 10, 635–647 (2007).
Acknowledgements
We thank the NERC, the Leverhulme Trust and the Royal Society for funding this research. We also thank S. Hughes of the Centre for Ecology and Hydrology for providing data and field assistance.
Author information
Authors and Affiliations
Contributions
N.F and C.F. designed the study; N.F. conducted the research; N.F. analysed the data and wrote the manuscript with C.F.
Corresponding author
Ethics declarations
Competing interests
The authors declare no competing interests.
Additional information
Peer review information Nature Climate Change thanks Tim Moore, Hongjun Wang and the other, anonymous, reviewer(s) for their contribution to the peer review of this work.
Publisher’s note Springer Nature remains neutral with regard to jurisdictional claims in published maps and institutional affiliations.
Supplementary information
Supplementary Information
Supplementary Figs. 1–3, Texts 1–4 and Tables 1–4.
Source data
Source Data Fig. 1
Numerical data used to generate graphs in Fig. 1a,b.
Source Data Fig. 2
Numerical data used to generate graphs in Fig. 2a,b.
Source Data Fig. 3
Numerical data used to generate graphs in Fig. 3a–c.
Source Data Fig. 4
Numerical data used to generate graphs in Fig. 4a,b.
Source Data Fig. 5
Numerical data used to generate graphs in Fig. 5a–c.
Rights and permissions
About this article
Cite this article
Fenner, N., Freeman, C. Woody litter protects peat carbon stocks during drought. Nat. Clim. Chang. 10, 363–369 (2020). https://doi.org/10.1038/s41558-020-0727-y
Received:
Accepted:
Published:
Issue Date:
DOI: https://doi.org/10.1038/s41558-020-0727-y
This article is cited by
-
Climate warming and elevated CO2 alter peatland soil carbon sources and stability
Nature Communications (2023)
-
Changes in organic matter properties and carbon chemical stability in surface soils associated with changing vegetation communities in permafrost peatlands
Biogeochemistry (2023)
-
Sugars dominate the seagrass rhizosphere
Nature Ecology & Evolution (2022)
-
Long-Term Carbon Accumulation in Temperate Swamp Soils: a Case Study from Greenock Swamp, Ontario, Canada
Wetlands (2022)
-
Vegetation and microbes interact to preserve carbon in many wooded peatlands
Communications Earth & Environment (2021)