Abstract
Matrix-assisted laser desorption–ionization mass spectrometry imaging in transmission-mode geometry (t-MALDI–MSI) can provide molecular information with a pixel size of 1 µm and smaller, which makes this label-free method highly interesting for characterizing the chemical composition of tissues and cells on a (sub)cellular level. However, a major hindrance for wider use of the technology is the reduced ion abundance at small pixel sizes. Here we mitigate this problem by use of laser-induced post-ionization (MALDI-2) and by adapting a t-MALDI-2 ion source to an Orbitrap mass analyzer. We demonstrate the crucial sensitivity and accuracy boosts that are achieved with this combination by visualizing the distribution of numerous phospho- and glycolipids in mouse cerebellum and kidney slices, and in cultured Vero B4 cells. With brain tissue, a pixel size of 600 nm was achieved. Our method could constitute a valuable new tool for research in cell biology and biomedicine.
This is a preview of subscription content, access via your institution
Access options
Access Nature and 54 other Nature Portfolio journals
Get Nature+, our best-value online-access subscription
$29.99 / 30 days
cancel any time
Subscribe to this journal
Receive 12 print issues and online access
$259.00 per year
only $21.58 per issue
Buy this article
- Purchase on Springer Link
- Instant access to full article PDF
Prices may be subject to local taxes which are calculated during checkout





Similar content being viewed by others
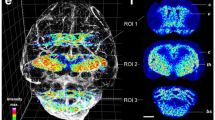
Data availability
The data that support the findings of this study, in particular all MSI data as presented in the main text, are available to download in the vendor neutral imzML format from https://uni-muenster.sciebo.de/s/bDrohQ7xJi7ZMIK or from the corresponding author on request. Selected MSI data were also uploaded to the METASPACE platform38 for visualization and annotation (https://metaspace2020.eu/project/Niehaus-2019-Transmission-MALDI-2).
References
Buchberger, A. R., DeLaney, K., Johnson, J. & Li, L. Mass spectrometry imaging: a review of emerging advancements and future insights. Anal. Chem. 90, 240–265 (2018).
Norris, J. L. & Caprioli, R. M. Analysis of tissue specimens by matrix-assisted laser desorption/ionization imaging mass spectrometry in biological and clinical research. Chem. Rev. 113, 2309–2342 (2013).
Soltwisch, J. et al. Mass spectrometry imaging with laser-induced postionization. Science 348, 211–215 (2015).
Kompauer, M., Heiles, S. & Spengler, B. Atmospheric pressure MALDI mass spectrometry imaging of tissues and cells at 1.4-μm lateral resolution. Nat. Methods 14, 90 (2017).
Spraggins, J. M. et al. Next-generation technologies for spatial proteomics: integrating ultra-high speed MALDI-TOF and high mass resolution MALDI FTICR imaging mass spectrometry for protein analysis. Proteomics 16, 1678–1689 (2016).
Kettling, H. et al. MALDI mass spectrometry imaging of bioactive lipids in mouse brain with a synapt G2-S mass spectrometer operated at elevated pressure: improving the analytical sensitivity and the lateral resolution to ten micrometers. Anal. Chem. 86, 7798–7805 (2014).
Ellis, S. R. et al. Automated, parallel mass spectrometry imaging and structural identification of lipids. Nat. Methods 15, 515–518 (2018).
Ellis, S. R., Soltwisch, J., Paine, M. R. L., Dreisewerd, K. & Heeren, R. M. A. Laser post-ionisation combined with a high resolving power orbitrap mass spectrometer for enhanced MALDI-MS imaging of lipids. Chem. Commun. 53, 7246–7249 (2017).
Zavalin, A., Yang, J. & Caprioli, R. Laser beam filtration for high spatial resolution MALDI imaging mass spectrometry. J. Am. Soc. Mass Spectrom. 24, 1153–1156 (2013).
Ogrinc Potočnik, N., Porta, T., Becker, M., Heeren, R. M. A. & Ellis, S. R. Use of advantageous, volatile matrices enabled by next-generation high-speed matrix-assisted laser desorption/ionization time-of-flight imaging employing a scanning laser beam. Rapid Commun. Mass Spectrom. 29, 2195–2203 (2015).
Belov, M. E. et al. Design and performance of a novel interface for combined matrix-assisted laser desorption ionization at elevated pressure and electrospray ionization with orbitrap mass spectrometry. Anal. Chem. 89, 7493–7501 (2017).
Hillenkamp, F., Unsöld, E., Kaufmann, R. & Nitsche, R. A high-sensitivity laser microprobe mass analyzer. Appl. Phys. Mater. Sci. Process 8, 341–348 (1975).
Vaeck, L. V., Struyf, H., Roy, W. V. & Adams, F. Organic and inorganic analysis with laser microprobe mass spectrometry. Part I: instrumentation and methodology. Mass Spectrom. Rev. 13, 189–208 (1994).
Vaeck, L. V., Struyf, H., Roy, W. V. & Adams, F. Organic and inorganic analysis with laser microprobe mass spectrometry. Part II: applications. Mass Spectrom. Rev. 13, 209–232 (1994).
Zavalin, A. et al. Direct imaging of single cells and tissue at sub-cellular spatial resolution using transmission geometry MALDI MS. J. Mass Spectrom. 47, 1473–1481 (2012).
Zavalin, A., Yang, J., Hayden, K., Vestal, M. & Caprioli, R. M. Tissue protein imaging at 1 μm laser spot diameter for high spatial resolution and high imaging speed using transmission geometry MALDI TOF MS. Anal. Bioanal. Chem. 407, 2337–2342 (2015).
Dreisewerd, K. The desorption process in MALDI. Chem. Rev. 103, 395–425 (2003).
Chu, K. Y. et al. Thermal proton transfer reactions in ultraviolet matrix-assisted laser desorption/ionization. J. Am. Soc. Mass Spectrom. 25, 310–318 (2014).
Gunzer, F., Krüger, S. & Grotemeyer, J. Photoionization and photofragmentation in mass spectrometry with visible and UV lasers. Mass Spectrom. Rev. 38, 202–217 (2019).
Bednařík, A., Bölsker, S., Soltwisch, J. & Dreisewerd, K. An on-tissue Paternò–Büchi reaction for localization of carbon–carbon double bonds in phospholipids and glycolipids by matrix-assisted laser-desorption-ionization mass-spectrometry imaging. Angew. Chem. Int. Ed. Engl. 57, 12092–12096 (2018).
Spivey, E. C., McMillen, J. C., Ryan, D. J., Spraggins, J. M. & Caprioli, R. M. Combining MALDI-2 and transmission geometry laser optics to achieve high sensitivity for ultra-high spatial resolution surface analysis. J. Mass Spectrom. 54, 366–370 (2019).
Steven, R. T. et al. Construction and testing of an atmospheric-pressure transmission-mode matrix assisted laser desorption ionisation mass spectrometry imaging ion source with plasma ionisation enhancement. Anal. Chim. Acta 1051, 110–119 (2019).
Fuchs, B. et al. Phosphatidylcholines and -ethanolamines can be easily mistaken in phospholipid mixtures: a negative ion MALDI-TOF MS study with 9-aminoacridine as matrix and egg yolk as selected example. Anal. Bioanal. Chem. 395, 2479–2487 (2009).
Sugiura, Y. et al. Visualization of the cell-selective distribution of PUFA-containing phosphatidylcholines in mouse brain by imaging mass spectrometry. J. Lipid Res. 50, 1776–1788 (2009).
Boggs, J. M., Gao, W. & Hirahara, Y. Myelin glycosphingolipids, galactosylceramide and sulfatide, participate in carbohydrate–carbohydrate interactions between apposed membranes and may form glycosynapses between oligodendrocyte and/or myelin membranes. Biochim. Biophys. Acta 1780, 445–455 (2008).
Chan, R. B. et al. Comparative lipidomic analysis of mouse and human brain with alzheimer disease. J. Biol. Chem. 287, 2678–2688 (2012).
Li, M. O., Sarkisian, M. R., Mehal, W. Z., Rakic, P. & Flavell, R. A. Phosphatidylserine receptor is required for clearance of apoptotic cells. Science 302, 1560–1563 (2003).
Schuhmann, K. et al. Intensity-independent noise filtering in FT MS and FT MS/MS spectra for shotgun lipidomics. Anal. Chem. 89, 7046–7052 (2017).
Amantonico, A., Urban, P. L., Fagerer, S. R., Balabin, R. M. & Zenobi, R. Single-cell MALDI-MS as an analytical tool for studying intrapopulation metabolic heterogeneity of unicellular organisms. Anal. Chem. 82, 7394–7400 (2010).
Schober, Y., Guenther, S., Spengler, B. & Römpp, A. Single cell matrix-assisted laser desorption/ionization mass spectrometry imaging. Anal. Chem. 84, 6293–6297 (2012).
Lanni, E. J., Rubakhin, S. S. & Sweedler, J. V. Mass spectrometry imaging and profiling of single cells. J. Proteom. 75, 5036–5051 (2012).
Patterson, N. H., Tuck, M., Van de Plas, R. & Caprioli, R. M. Advanced registration and analysis of MALDI imaging mass spectrometry measurements through autofluorescence microscopy. Anal. Chem. 90, 12395–12403 (2018).
Schramm, T. et al. imzML—a common data format for the flexible exchange and processing of mass spectrometry imaging data. J. Proteom. 75, 5106–5110 (2012).
Pauling, J. K. et al. Proposal for a common nomenclature for fragment ions in mass spectra of lipids. PLoS ONE 12, e0188394 (2017).
Fahy, E., Sud, M., Cotter, D. & Subramaniam, S. LIPID MAPS online tools for lipid research. Nucleic Acids Res. 35, W606–W612 (2007).
Korf, A., Jeck, V., Schmidt, R., Helmer, P. & Hayen, H. Lipid species annotation at double bond position level with custom databases by extension of the MZmine 2 open-source software. Anal. Chem. 91, 5098–5105 (2019).
Liebisch, G. et al. Shorthand notation for lipid structures derived from mass spectrometry. J. Lipid Res. 54, 1523–1530 (2013).
Palmer, A. et al. FDR-controlled metabolite annotation for high-resolution imaging mass spectrometry. Nat. Methods 14, 57–60 (2017).
Acknowledgements
We thank N. Schwab (Institute for Translational Neurology), S. Albrecht (Institute of Neuropathology) and T. Weide (Molecular Necrophology) for providing mouse brain and kidney samples and for help with data interpretation; J. Müthing, N. Skutta, D. Mense for cell culturing; H. Nüsse for SEM images; W. Brock and Tascon GmbH for profilometer measurements; C. Bookmeyer, G. Pohlentz and M. Mormann for assistance with lipid assignments; M. Wiese for programming a software module for steering the piezo stage; A. Korf for expert assistance with MZmine software; W. Kramer (Fotozentrale of the University Hospital Münster) for design and production of Fig. 1; and members of the central fine-mechanical workshop of the Münster Medical School for help building the t-MALDI stage. Financial support by the German Research Foundation (DFG; grants DR 416/12-1, DR 416/13-1, SO 976/3-1 and SO 976/4-1) and the Interdisciplinary Center for Clinical Research (IZFK; grant Drei2/018/17) is gratefully acknowledged.
Author information
Authors and Affiliations
Contributions
M.N., J.S. and K.D. conceived the experiments; M.N. and J.S. designed the t-MALDI ion source; M.B. provided crucial input for the adaptation with the dual-ion funnel injector and the Orbitrap mass analyzer; M.N. and J.S. conducted the experiments; M.N., J.S. and K.D. performed the data analysis; K.D. and J.S. provided funds; and M.N., J.S. and K.D. wrote the paper.
Corresponding author
Ethics declarations
Competing interests
M.N., J.S. and K. D. declare no competing interests. M.B. is a general manager of Spectroglyph LLC, the company that developed and made commercially available the dual-funnel MALDI–ESI Injector interface.
Additional information
Peer review information: Rita Strack was the primary editor on this article and managed its editorial process and peer review in collaboration with the rest of the editorial team.
Publisher’s note: Springer Nature remains neutral with regard to jurisdictional claims in published maps and institutional affiliations.
Integrated supplementary information
Supplementary Figure 1 Overview bright field image of the mouse brain section evaluated in Figs. 2, 4 and Supplementary Fig. 3.
Overview bright field image of the mouse cerebellum tissue section that was used to generate the ion images of Fig. 2, Fig. 4, and Supplementary Fig. 2, respectively. The adjacent areas that were analyzed for producing the ion images are indicated by the black frames. For Fig. 2, the big rectangle to the right denotes the positive ion mode measurements whereas the negative ion mode measurements (left) were conducted on three separate days. The HCCA matrix-coated tissue sample remained in the t-MALDI ion source during the full experimental circle, without that this caused any notable degradation in analytical performance.
Supplementary Figure 2 Comparison of t-MALDI with t-MALDI-2.
(a) t-MALDI (black trace, top panel) and t-MALDI-2 (red trace, bottom) mass spectra acquired from mouse cerebellum in the positive ion mode at 1 µm pixel size. HCCA was used as a matrix. (b) Bright field image of the areas across which the mass spectra were acquired, the microscopic image was taken prior to spray-coating the HCCA MALDI matrix. (c) Ion images of selected lipids acquired in the t-MALDI (top) and t-MALDI-2 modes (bottom). Comparable experiments from four different mouse brain samples yielded similar results.
Supplementary Figure 3 Comparison of [DAG(36:1)]+- and putative PC(36:1)-precursor-derived ion images.
Ion images of four most prominent 36:1-phospholipid ion signals detected from mouse cerebellum by t-MALDI-2 in the positive ion mode and that of the [DAG(36:1)]+ dissociation product. The same data set as used for producing Fig. 2 of the main text was evaluated. (a) m/z 605.552, [DAG(36:1)]+; (b) m/z 703.526, PA(36:1); (c) m/z 729.542, PC(36:1)-N(CH3)3 or isobaric PA(38:2); (d) 746.469; PE(36:1); (d) m/z 788.616, PC(36:1); (f) m/z 790.559, PS(36:1). All ions were detected as [M + H]+ species. All ‘Viridis’ false color pallets were adjusted to optimize the presentation of the individual ion images; hence, they are not to scale if the images were to be compared among each other. Comparable experiments from four different mouse brain samples yielded similar results.
Supplementary Figure 4 MSI and light microscopy data of mouse kidney tissue—part 1 and part 2.
(a) Microscopic image of a 6 µm-thick, H&E-stained section of a mouse kidney tissue. The stain was produced following the t-MALDI measurements and removing the matrix by a washing step. The black rectangular area indicates the approximate regions with which t-MALDI-2–MSI experiments were conducted. (b) Autofluorescence image taken of the analyzed section after the MSI measurement. (c to f) Representative t-MALDI-2–MS images acquired in the positive ion mode: (c) m/z 768.544, PE(38:4)+, (d) m/z 724.524, PE(O-36:5)/PE(P-36:4); (e) m/z 792.555, PE(40:6), (f) m/z 644.502, CerP(36:1-OH; presumably CerP(d18:1/C18:0-)). All ions were detected as [M + H]+ species. (g) Mass spectrum averaged over 580,000 pixels. Only a few selected major ion signals are annotated based on accurate mass. A more complete assignment of the detected lipids is provided in Supplementary Table 3. HCCA was used as the MALDI matrix and prepared using a spraying protocol. The ablation laser was focused to an effective spot size of about 1.3 µm by diameter and the step size of the piezo stage was set to 1.2 µm. Comparable experiments from one different mouse kidney sample yielded similar results.
Supplementary Figure 5 Single pixel MS/MS data recorded at 1 µm pixel size.
(a) Bright-field microscopic image of a 6 µm-thick section of a mouse brain tissue before matrix coating. (b) t-MALDI-2-MS/MS images of diagnostic fragments recorded from the same section following spray-coating with HCCA matrix. (c) Single pixel MS/MS spectrum of GalCer(d18:1/C22:1), m/z of the [M + H]+ precursor, 810.681; (d) MS/MS spectrum of PE(40:6), m/z of the [M + H]+ precursor, 792.554, recorded from 4 adjacent pixels, and (e) MS/MS spectrum of PS(40:6), m/z of the [M + H]+ precursor, 836.543, also recorded from 4 pixel. Experiments were conducted on a single sample only.
Supplementary Figure 6 SEM images of brain sections coated with HCCA matrix before and following t-MALDI-2-MSI.
(a) SEM image of a 6 µm-thick mouse brain tissue section, spray-coated with HCCA matrix according to the protocol outlined in the Methods section. The displayed region corresponds to the white matter of mouse cerebellum. (b) Ablation crater pattern formed during a t-MALDI-2-MSI experiment and use of 25 laser pulses. The t-MALDI-MSI experiment was conducted at a step size of 5.0 µm. (c) Enlarged view into the irradiated sample. Experiments were conducted on a single sample only.
Supplementary Figure 7 Tandem-MS data to demonstrate adduct formation with indium cations.
An example tandem MS spectrum of an (unknown) analyte species with an indium adduct. Experiments were conducted on a single sample only.
Supplementary Figure 8 Bright-field and t-MALDI-2-MSI-data of cultured Vero B4 cells in the negative ion mode.
Bright field and exemplary ion images of a Vero B4 cell culture imaged by t-MALDI-2-MSI in the negative ion mode (cf. Fig. 5 of the main text for positive ion mode measurements). (a) Bright field image of sub-confluently grown cells with the DHB matrix applied by sublimation; the depicted area displays the region from which the ion images in panels b to d were recorded. (b) M/z 742.538, PE(36:2); (c) m/z 788.545, PS(36:1); (d) m/z 861.550, PI(36:2); all ions were detected as [M–H]− species. A more complete list of tentatively annotated lipids is provided in Supplementary Table 4. Comparable experiments from one different mouse kidney sample yielded similar results.
Supplementary Figure 9 Snapshots of the t-MALDI setup.
Snapshots of the custom-build t-MALDI-2 ion source. (a) The following components are depicted: 1- telescope lenses for beam manipulation. One of the lenses is attached to a piezo stage for fine adjustment of the laser focus on the sample slide; 2- dielectric mirror in front of the quartz window guiding the laser beam into the ion source; 3- UV-grade mirrors; 4- focusing lens for the CCD camera. (b) The interior components of the ion source. 1- 50x magnifying UV-grade microscope objective; 2- piezo sample stage; 3- ITO coated slide holder; 4- constant force spring to account for the weight of the attached sample holder and PEEK bracelet.
Supplementary information
Supplementary Information
Supplementary Figs. 1–9 and Supplementary Tables 1–5
Rights and permissions
About this article
Cite this article
Niehaus, M., Soltwisch, J., Belov, M.E. et al. Transmission-mode MALDI-2 mass spectrometry imaging of cells and tissues at subcellular resolution. Nat Methods 16, 925–931 (2019). https://doi.org/10.1038/s41592-019-0536-2
Received:
Accepted:
Published:
Issue Date:
DOI: https://doi.org/10.1038/s41592-019-0536-2
This article is cited by
-
Multiplex protein imaging in tumour biology
Nature Reviews Cancer (2024)
-
Spatial analysis of the osteoarthritis microenvironment: techniques, insights, and applications
Bone Research (2024)
-
Nanomaterial Assisted Exosome Analysis Using Mass Spectrometry
Chemical Research in Chinese Universities (2024)
-
A critical evaluation of ultrasensitive single-cell proteomics strategies
Analytical and Bioanalytical Chemistry (2024)
-
Spatial probabilistic mapping of metabolite ensembles in mass spectrometry imaging
Nature Communications (2023)